STRUCTURES Blog > Posts > The Curious Case of the World’s Smallest Fluid
The Curious Case of the World’s Smallest Fluid
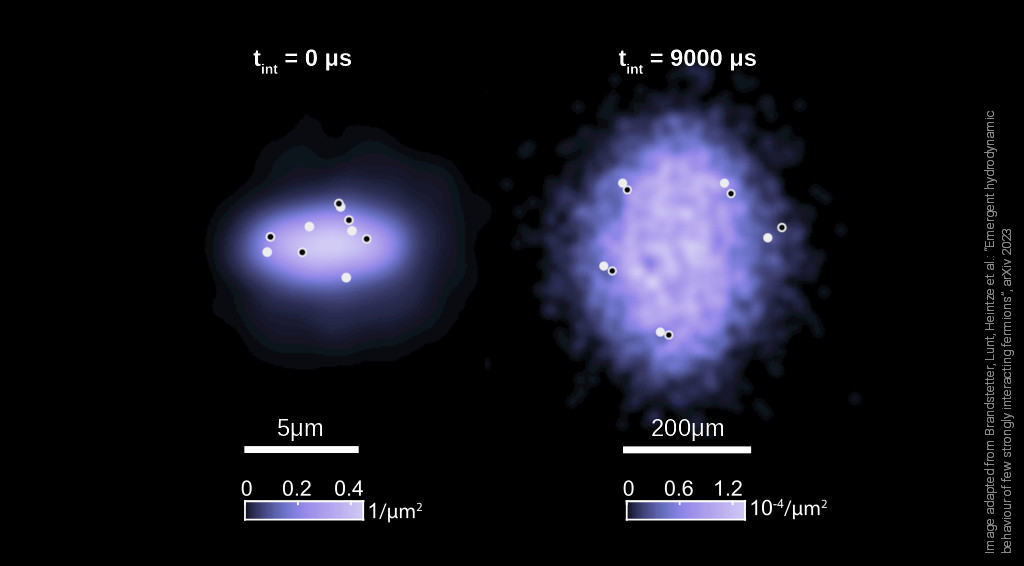
Editor’s note: This blog post is based on the talk “Eins, zwei, viele – Die kleinste Flüssigkeit der Welt” given by Sandra Brandstetter & Philipp Lunt at the public lecture series event “Akademische Mittagspause 2023: Strukturen in der Welt” (German). The content was edited by the authors together with the STRUCTURES Blog Team, after the transcript has been auto-generated and edited with the support of artificial intelligence tools.
The natural world never ceases to fascinate us with its myriad of complex patterns and phenomena, one of which is collective behaviour. Groups of particles, objects or even living organisms interact and move in astonishingly coordinated ways, defying the conventional rules of individuality. To understand the peculiar phenomena of collective behaviour, scientists have turned their attention to diverse systems, ranging from bacterial colonies to bird flocks.
The sight of a flock of birds in perfect synchrony presents a particularly captivating example of emergent behaviour. Countless individual motions of birds join to form a coherent collective movement of the flock. To truly grasp the essence of this phenomenon, we need to zoom out and observe the flock as a whole entity, rather than fixating on individual birds. Close up, the motions of each bird may appear random, uncorrelated and devoid of any apparent coordination. If we removed one bird, would the behaviour of the flock change? Most likely not - however, what if we continued the game by removing more and more birds until only one remains? Our description of collective behaviour would break down, instead, we would have to return to modelling the single-bird behaviour.
Let us now apply that concept to liquids – vast collections of quadrillions of molecules. In such liquids, complex dynamics unfold already in simple situations, e.g. when a single water droplet falls into a liquid. Even though the drop of water consists of quadrillions of molecules interacting with each other in a complex interplay, its behaviour can be described as a whole, disregarding the behaviour of each individual molecule. But what if we look at systems comprising only a few particles? Can these small systems mirror the complexities of a fluid, displaying similar collective behaviour on a minuscule scale? In other words: How many particles does it take to form a liquid?
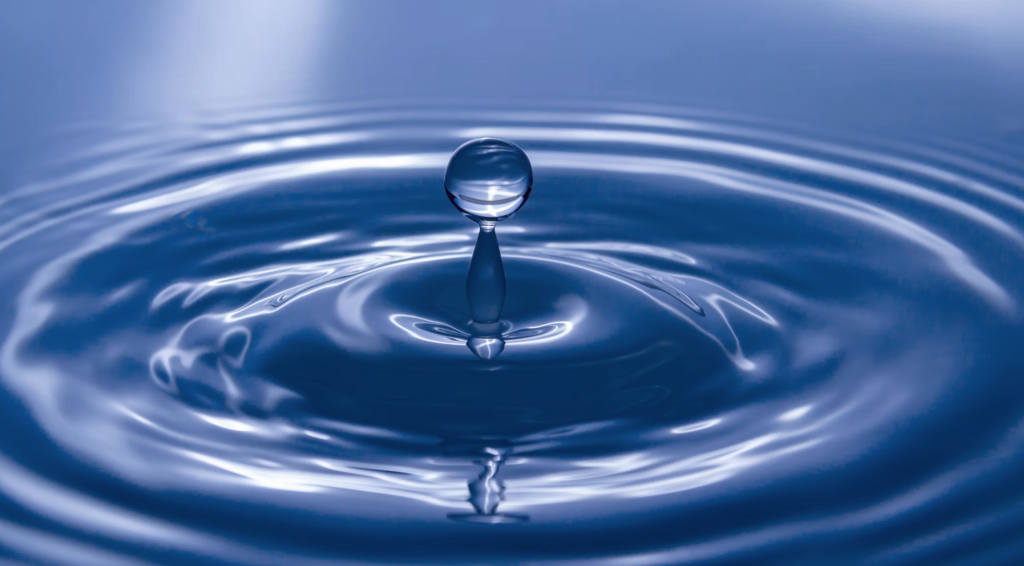
From Liquids to Ultracold Atoms – Entering the Strange Realm of the Quantum World
In our laboratories, we can investigate ultracold lithium atoms. These atoms are cooled down to just a few billionth degrees above absolute zero. At these temperatures, quantum effects like Heisenberg’s uncertainty principle manifest - it becomes impossible to precisely determine both the momentum and position of individual particles simultaneously. This fundamental limitation leads to delocalization, where the atoms spread out and exhibit wave-like characteristics. Another intriguing property arises at these low temperatures – the atoms become indistinguishable. In other words, one cannot discern one atom from another, making it impossible to label them as atom 1, atom 2, and so on.
Even though these concepts may sound abstract, in our research we are able to control and manipulate systems such as ultracold lithium atoms using magnetic fields and lasers. Through careful manipulation, it is possible to precisely control the number of atoms that are present in the experiment. Using cutting-edge imaging techniques, we are able to image single atoms. However, due to the rules of quantum mechanics, each particle doesn’t have a single exact position, but rather a given probability for being found at a certain position. Consequently, we need to analyze and process thousands of individual images, allowing us to reconstruct this probability distribution linked to the particle’s quantum-mechanical wave function.
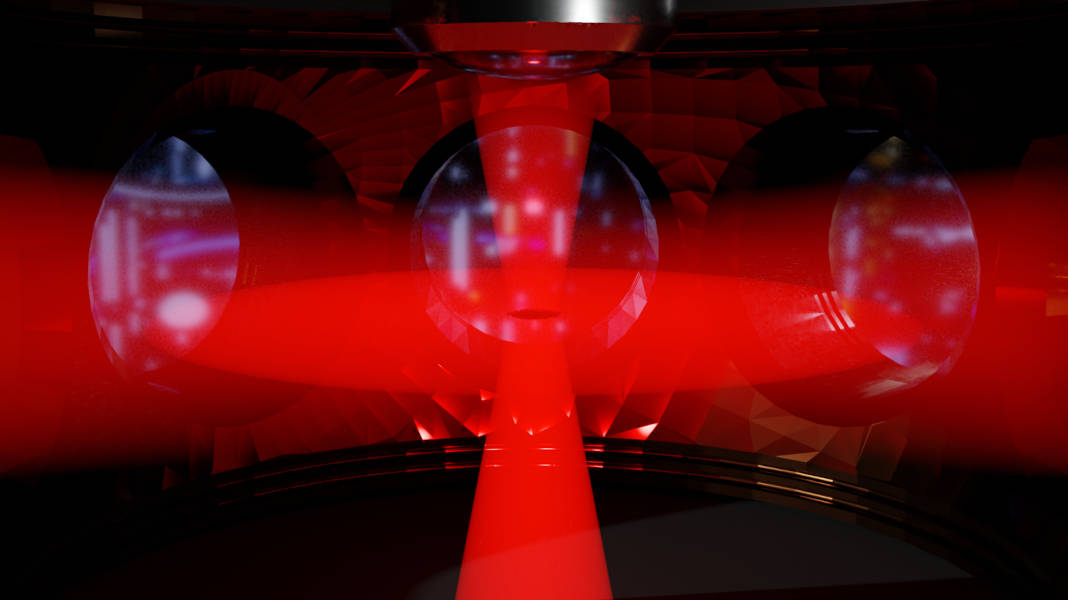
Exploring the Quantum World with Cold Atom Experiments
What Are Cold Atom Experiments?
Cold atom experiments are a fascinating branch of modern physics that allows scientists to explore the bizarre and counterintuitive world of quantum mechanics in a controlled laboratory setting. These experiments involve cooling atoms to ultra-low temperatures, typically just a few billionths of a degree above absolute zero (-273.15°C), using sophisticated techniques that have earned researchers Nobel Prizes. Cold atoms are well isolated systems, making them ideal candidates for investigating the quantum behaviour of matter.
Why Fermionic Systems Matter
Fermionic systems are a specific type of quantum system that obey the Pauli exclusion principle, a fundamental concept in quantum physics. This principle states that no two fermions (such as electrons or certain types of atoms) can occupy the same quantum state simultaneously. Understanding fermionic systems is crucial in various scientific domains, from understanding the electronic structure of materials to exploring exotic states of matter like superconductivity.
Key Components of Cold Atom Experiments
Laser Cooling: One of the primary techniques used in cold atom experiments is laser cooling. Shining carefully tuned lasers on a cloud of atoms causes the atoms to slow down and reach extremely low temperatures.
Optical Traps: After laser cooling, optical traps are used to hold and manipulate the cold atoms. These traps are precisely focused laser beams that create regions of high intensity where atoms are trapped. Within these traps, we cool the atoms even further using a process called evaporative cooling. By gradually lowering the intensity of the trap, allowing the atoms with highest energy to escape, we cool the remaining atoms to even lower temperatures, creating conditions necessary for the study of exotic quantum phenomena.
Feshbach Resonances: One of the remarkable features of cold atom experiments, particularly those involving fermionic systems, is the utilization of Feshbach resonances. Feshbach resonances are a phenomenon where the interactions between atoms can be dramatically altered by applying a magnetic field at a specific strength. This magnetic tuning knob allows us to precisely control the scattering properties of atoms and create a wide range of intriguing quantum states.
Bose-Einstein Condensates (BECs): In addition to fermionic systems, researchers also create Bose-Einstein condensates, which are another exotic state of matter. BECs are formed when bosons (particles that can occupy the same quantum state) cool to near absolute zero and collapse into a single quantum state, exhibiting quantum phenomena on a macroscopic scale.
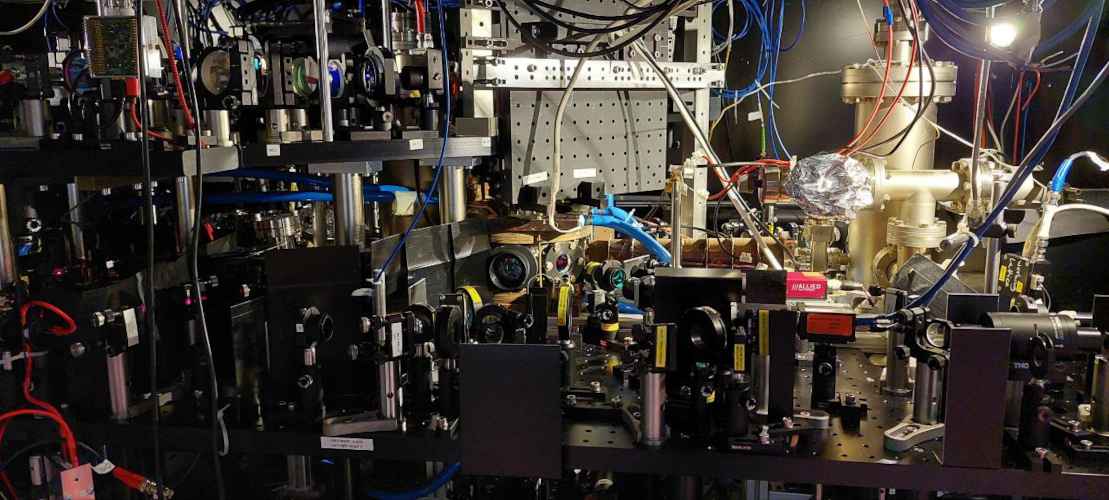
A Fluid Made of 10 Atoms?
In the controlled environment of our lab, we are able to explore whether fluid-like behaviour can manifest with just a few atoms. A fluid exhibits a distinct dynamical behaviour when released from an elliptically shaped confining container - expanding faster in the tightly confined direction, which leads to an inversion of the initial ellipticity. If we can reproduce this deformation with only a small number of atoms in our lab, it would signify the presence of fluid characteristics.
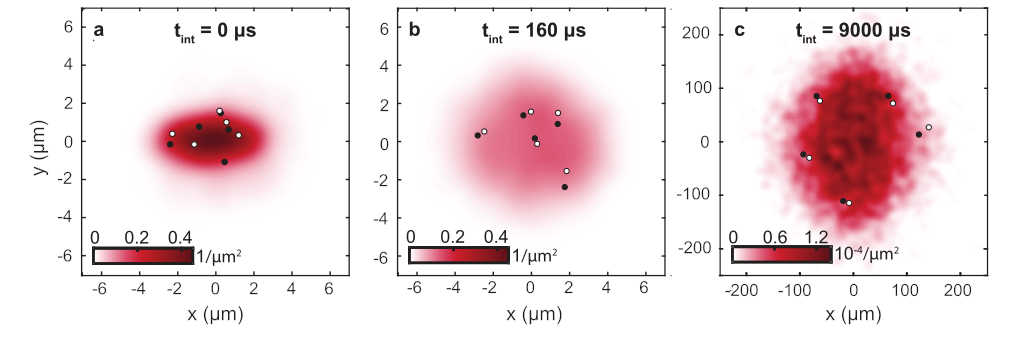
The image displayed in Fig. 4 represents the quantum-mechanical probability density distribution for a system of 10 particles. As indicated by the scales, the system size is around 10 times smaller than an average human hair. As we let the atoms expand over time, we can indeed witness the system accelerating faster along one direction, interchanging the ellipse’s major axes. This deformation arises from the faster pressure drop in one direction, propelling the particles outward. The essence of this deformation bears striking resemblance to what is typically seen in fluid systems containing a large number of particles. It is astonishing that reducing the atom count to a mere 10 is sufficient to maintain fluid-like behaviour, showcasing the persistence of the phenomena we typically associate with systems containing quadrillions of particles.
This revelation challenges our conventional understanding of collective behaviour, as it indicates that the essence of fluid-like motion lies in the cooperative interactions between particles, not their sheer number. This breakthrough opens new doors for exploring the fascinating intricacies of emergent behaviour in quantum systems.
Conclusion
Our experiments have demonstrated that even with a few atoms, one can detect signatures of fluid-like behaviour. The effects observed echo those found in conventional fluid systems with a vast number of particles, yet they have been observed in a small system of ten ultracold atoms. The quantum nature and delocalization of our atoms causes the collective behaviour at low numbers, in contrast to the classical case where one would not describe few birds as a flock. These findings contribute and pave the way to a deeper understanding of collective phenomena in quantum systems, offering valuable insights into the dynamic behaviours of fluids on both macroscopic and microscopic scales.
Further reading
- Original research article (preprint): Sandra Brandstetter, Philipp Lunt, Carl Heintze, Giuliano Giacalone, Lars H. Heyen, Maciej Gałka, Keerthan Subramanian, Marvin Holten, Philipp M. Preiss, Stefan Floerchinger, Selim Jochim: “Emergent hydrodynamic behaviour of few strongly interacting fermions” (arXiv:2308.09699)
Tags:
Ultracold Atoms
Physics
Quantum Physics
Fermions
Fluids
Collective Phenomena
Correlations
Uncertainty
Probability